Colorado Animal Specialty & Emergency (CASE)
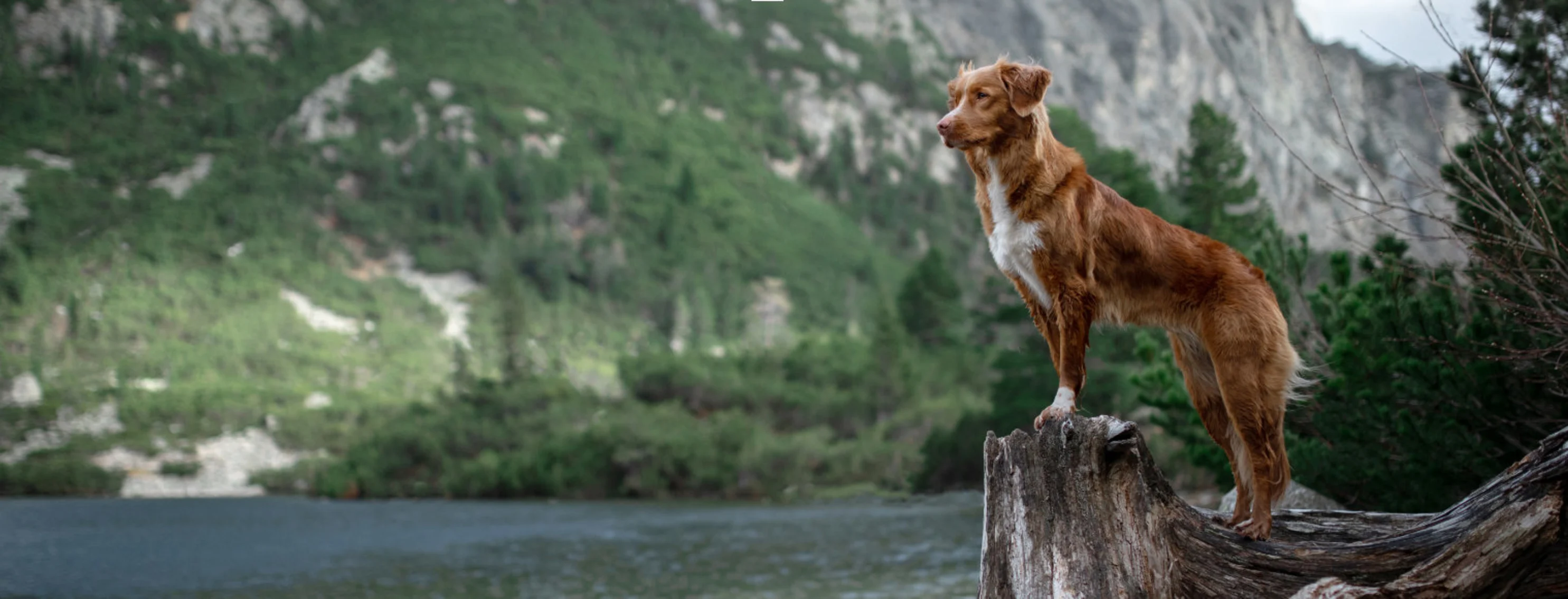
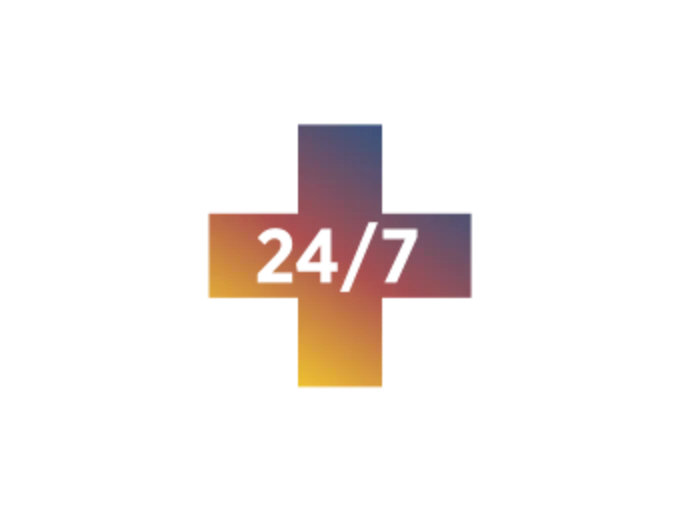
24/7 Emergency
We provide around-the-clock emergency and critical care to dogs and cats 365 days a year in the greater Boulder area.
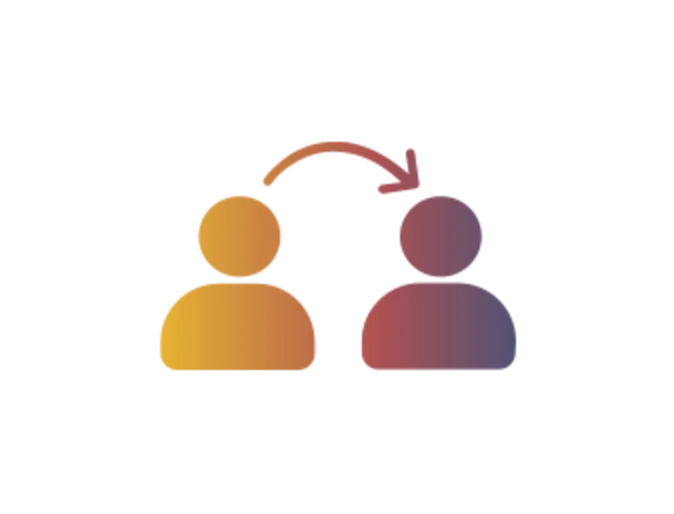
Referrals
Are you a veterinarian looking to submit a referral?
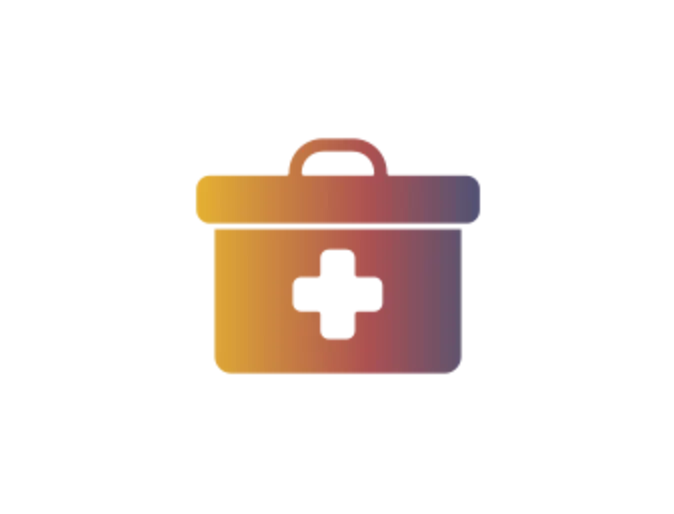
Join Our Team
Interested in learning more about career opportunities at CASE?